
Yi Cui is harnessing the power of nanoscience to grow extremely small structures—which play a huge role in the clean energy transition
In a wrestling match between a pygmy mouse lemur and a gorilla, intuition suggests the larger primate would win. The notion that size equals strength also finds resonance in science fiction, depicted in works like the 1956 novel The Shrinking Man and the 1989 film Honey, I Shrunk the Kids, both exploring how terrifying the world would be if humans were suddenly smaller than ants.
Nanoscience turns this convention on its head: As materials decrease in size to the nanoscale, they can actually exhibit increased strength. How large is one nanometer? One billionth of a meter, or roughly how much your fingernails grow in one second. The thickness of a single sheet of paper measures a staggering 100,000 nanometers.
Yi Cui, the Fortinet Founders Professor of Materials Science and Engineering, has dedicated nearly two decades to unlocking nanoscience’s potential to revolutionize a pivotal aspect of the clean energy transition: battery storage.

While lithium-ion batteries are commonly associated with portable devices—cell phones, pacemakers—demand for energy-dense batteries is growing in the world of decarbonization. The transition to electric cars and planes, critical for reducing fossil fuel dependence, hinges on developing powerful batteries. And as more households and businesses adopt solar power, there’s an escalating need for large, energy-dense batteries capable of storing excess power for use overnight or during adverse weather conditions.
Unlike fuel cells—another frontrunner in the clean energy transition—batteries offer the advantage of leveraging the existing electricity infrastructure. But they also present challenges, namely safety and cost. Any viable battery solution must withstand all possible temperature conditions and be inexpensive enough for widespread adoption.
Enter nanoscience. The physical and chemical properties of materials can change dramatically at the nanoscale, driven in part by quantum mechanics and a greater surface area-to-volume ratio. For instance, while carbon at the macroscale could constitute, say, the snappable graphite in your pencil, carbon at the nanoscale is stronger than steel. Likewise, aluminum, which is stable in bulk, becomes combustible at the nanoscale. For Yi Cui, such radical changes at the nanoscale open up a path for groundbreaking innovation in battery technology.
Most batteries consist of positively and negatively charged conductors—an anode and cathode, respectively—suspended in an electrolyte. As ions move between the anode and the cathode, energy discharges, generating power.
Silicon has long been attractive as a potential anode because it has greater energy density and costs much less than the graphite anodes predominantly used in lithium-ion batteries. However, silicon’s volume increases 400 percent when lithium is inserted and extracted, destroying the battery.
Cui’s creative solution? Making the materials smaller. He used a vapor-liquid-solid (VLS) process to grow silicon nanowires, which involves exposing metal nanoparticle catalysts to silicon gas at temperatures from 400-500 degrees Celsius, dissolving silicon into nanoparticles until liquid droplets form.
“You keep adding silicon atoms to this droplet, and it will super-saturate and precipitate out in a solid silicon nanowire shape,” Cui says. “It’s a really beautiful, elegant mechanism to make these wires.”
These new silicon nanowire electrodes could take significant strain without the rapid degradation that occurs to silicon in bulk, allowing for many cycles of charging and discharging. Since silicon stores 10 times more lithium than graphite as an anode, this allows for nearly double the amount of energy in a full-size battery.
Cui published these findings in a landmark paper in 2008. In addition to showing it was possible to create a lithium-ion battery with a pure silicon anode, the paper effectively pioneered the field of nanoscience for energy storage.
Chasing the “holy grail” of energy storage
According to Cui, lithium metal batteries are the “holy grail” of battery research. They are the primary focus for the Battery500 Consortium, a group of researchers from national labs, academia, and industry that aims to increase the energy of batteries, allow for more charge/discharge cycles, and reduce battery cost—all crucial for achieving the Department of Energy’s goals for carbon-neutral energy and electrification. Cui, co-director of Battery500, says lithium metal offers even greater capacity than lithium-ion batteries with a silicon anode.
Cui spent years searching for an imaging tool that could offer insight into lithium metal and other battery materials. Since electron beams from electron microscopes destroy lithium metal, observing key features at the atomic scale was impossible. In particular, Cui wanted to examine lithium metal’s solid electrolyte interphase—a layer of material that forms between the anode and the liquid electrolyte.
When he was a postdoctoral scholar at Berkeley, Cui learned about cryo-electron microscopy (cryo-EM), a technology developed by structural biologists to study biomolecules such as proteins, but the spatial resolution was far from what was needed to investigate lithium metal. Ten years later, he realized that advances in cryo-EM technology could potentially revolutionize battery research.
Cui’s willingness to consider outside-the-box and outside-the-discipline approaches paid off. It took his lab only four months to develop a cryo-EM technique to image lithium metal. By cooling the material down to the temperature of liquid nitrogen, Cui was able to capture the first-ever images of lithium metal and its solid electrolyte interphase at the atomic scale. This high-resolution imaging shed light on the nature of lithium dendrites, which cause lithium metal batteries to short-circuit, even allowing Cui to measure the distance between atoms (a seventh of a nanometer).
“No one could believe it at the beginning!” laughs Cui, remembering how hard it was to convince peer reviewers for Science that these really were images of lithium metal.
“When I can’t find the solution, I just let the problem hang there. Then, I’ll think about it again a week or months later. And this can go on for decades,” says Cui. “But I do have an example where, a decade later, I finally figured it out.”

“
WHEN I CAN’T FIND THE SOLUTIONS, I JUST LET THE PROBLEM HANG THERE.
Then, I’ll think about it again a week or months later. And this can go on for decades. But I do have an example where, a decade later, I finally figured it out.”
Yi Cui
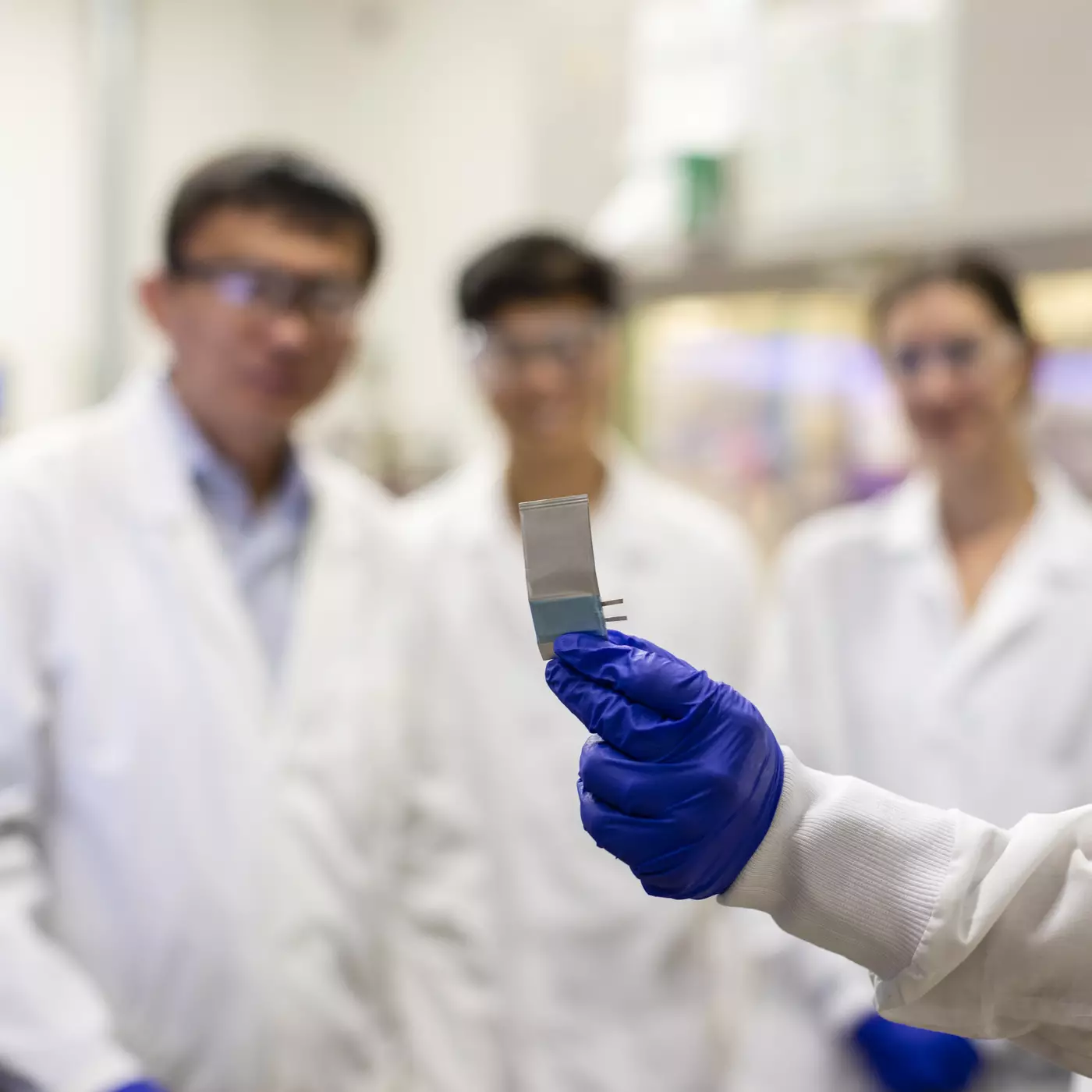
A battery prototype in Cui’s lab.
With the most challenging problems, Cui is willing to persevere and even enjoys doing so—a vital quality for a scientist confronting climate change.
“Of course, many people feel scared because the problem is so huge they worry there is no solution, and they become pessimistic,” he reflects. “I’m optimistic because I believe we will be able to find the solutions.”
Sustaining Life + Accelerating Solutions
Sustaining Life + Accelerating Solutions: The impact
Why it matters
Safe, inexpensive batteries with high energy density are essential to transition to clean energy. Cui’s research could help combat climate change by storing wind and solar energy, reducing dependence on fossil fuels, and meeting pivotal sustainability goals.
What’s next
In addition to his lab’s ongoing research, Cui will leverage his experience as an entrepreneur as the new director of Stanford’s Sustainability Accelerator, which aims to drive the translation of technology and policy solutions to the real world.
Why Stanford
Before Cui completed his postdoctoral fellowship at Berkeley, he had received about a dozen tenure-track job offers. Still, he knew he wanted to go to Stanford after his first interview on campus. He recognized the school’s unique, collaborative environment and its vital relationship to industry.
- SEO Powered Content & PR Distribution. Get Amplified Today.
- PlatoData.Network Vertical Generative Ai. Empower Yourself. Access Here.
- PlatoAiStream. Web3 Intelligence. Knowledge Amplified. Access Here.
- PlatoESG. Carbon, CleanTech, Energy, Environment, Solar, Waste Management. Access Here.
- PlatoHealth. Biotech and Clinical Trials Intelligence. Access Here.
- Source: https://genesisnanotech.wordpress.com/2024/03/26/stanford-universitys-battery-super-power/