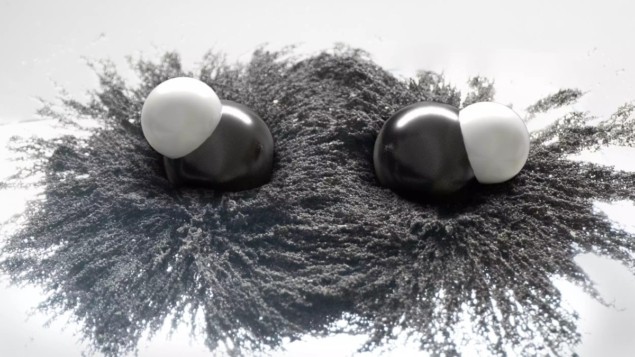
Weakly bound tetratomic molecules that are more than 3000 times colder than any previous four-atom molecules have been created using a newly developed “electroassociation” technique. The work, which is based on a 2003 proposal, could make it possible to assemble even larger molecules at ultracold temperatures, open up studies in superfluidity and superconductivity, and even find applications in quantum computing.
In 2003, theoretical physicist John Bohn of JILA in Boulder, Colorado was part of a team led by the renowned experimentalist Deborah Jin, who died in 2015. They were studying the effects of magnetic fields on ultracold fermionic gases. The researchers discovered that the atoms formed weakly bound diatomic molecules when they tuned the value of the field across a so-called Feshbach resonance at which the binding energy was equal to that of the molecules. This process subsequently became known as magnetoassociation.
Then, in 2008, a team led by Jin and her University of Colorado colleague Jun Ye demonstrated the conversion of these fragile dimers into ground-state molecules using a three-level laser cooling technique called stimulated Raman adiabatic passage (STIRAP). The two techniques have subsequently been used by countless other groups to create ultracold dimers for a plethora of applications such as the study of quantum chemistry.
Magnetoassociation only works, however, on particles with magnetic dipole moments – which means they must have unpaired electrons. Jin’s group was working with potassium atoms, which are magnetic. Once they associate to form diatomic potassium molecules, they no longer respond to magnetic fields.
Why not electroassociation?
In the same year, Bohn and colleague Aleksandr Avdeenkov published a theoretical paper suggesting that it might be possible to induce non-magnetic molecules to pair up if they had an electric dipole moment: “Magnetoassociation was something that existed, so we thought, well, why not electroassociation?” says Bohn, “We didn’t give it any more thought than that.”
In 2023, however, using a modified version of Bohn’s original proposal, Xin-Yu Luo of the Max Planck Institute for Quantum Optics in Germany and colleagues placed strongly bound, ultracold sodium potassium molecules (produced by magnetoassociation and STIRAP) in an oscillating external microwave field. At specific field values, they found spectroscopic evidence of a resonant state unlike anything previously seen between pairs of molecules. In this state the two molecules danced in parallel as their own electric dipole moments modified the applied potential. The resulting interaction was repulsive at short distances but attractive at long distances, resulting in a bound state that was about 1000 times larger than the diameters of the individual molecules. At the time, however, the researchers only had evidence that the state existed – not any controlled means to place particles into it.
Circularly polarized microwaves
In the new work, the Max Planck researchers and colleagues at Wuhan University in China found that, by applying a circularly polarized microwave field to sodium potassium molecules at temperatures around 100 nK before increasing the ellipticity of the field, they could induce some of them to form tetramers. The team also managed to dissociate the tetramers and, by looking at the shape of the dimers released, image the tetramer wavefunction. They describe this in Nature.
“The binding energy is radio-frequency scale,” says Luo, “It’s more than 10 orders of magnitude weaker than typical chemical bond energy.”
The researchers now hope to use STIRAP to create strongly bound tetramers. This will be no easy task, says Luo, because it requires a suitable intermediate energy level, and tetramers have many more energy levels than dimers. “Even for me it’s an open question whether we can find a suitable state in the forest of energy levels,” says Luo. If they can, however, it holds out the tantalizing possibility of repeating the technique to build ever-larger molecules.
Entangled molecules make a novel qubit platform
The researchers are also looking to cool their molecules further into a Bose–Einstein condensate (BEC). They would then become a powerful tool for studying the crossover between the BEC state and the Bardeen–Cooper–Schrieffer (BCS) state of superconductivity. This crossover is crucial to understanding high-temperature superconductivity. Such a tool would allow physicists to tune the constituents of the condensate between fermionic dimers and bosonic tetramers simply by tuning the microwave field. This would allow them to turn a BEC into a degenerate Fermi gas that supports Cooper pairs.
Further into the future, the system could even be useful in quantum computing as theoretical predictions suggest it should support topologically protected Majorana zero modes that could be used to create noise-resistant qubits.
Bohn describes the work of Luo and colleagues as fantastic, adding “Not only is it well done, but it’s something that a lot of people have been hoping for for a long time.” After reading the group’s 2023 paper, he collaborated with two colleagues to develop a theoretical framework, described in Physical Review Letters in July 2023, for achieving electroassociation based on the group’s results, and showing the ideal rate at which to alter the fields. “While we were doing that, they already did the experiment,” he says; “Evidently they figured that out just fine on their own.”
- SEO Powered Content & PR Distribution. Get Amplified Today.
- PlatoData.Network Vertical Generative Ai. Empower Yourself. Access Here.
- PlatoAiStream. Web3 Intelligence. Knowledge Amplified. Access Here.
- PlatoESG. Carbon, CleanTech, Energy, Environment, Solar, Waste Management. Access Here.
- PlatoHealth. Biotech and Clinical Trials Intelligence. Access Here.
- Source: https://physicsworld.com/a/ultracold-four-atom-molecules-are-bound-by-electric-dipole-moments/