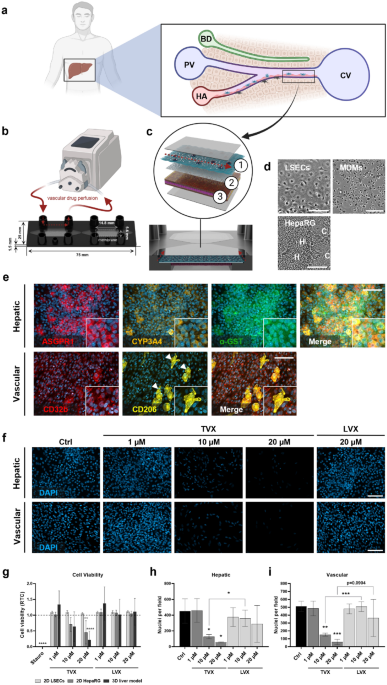
Stevens, J. L. & Baker, T. K. The future of drug safety testing: Expanding the view and narrowing the focus. Drug Discov. Today 14, 162–167. https://doi.org/10.1016/j.drudis.2008.11.009 (2009).
Watkins, P. B. Drug safety sciences and the bottleneck in drug development. Clin. Pharmacol. Ther. 89, 788–790. https://doi.org/10.1038/clpt.2011.63 (2011).
Wysowski, D. K. & Swartz, L. Adverse drug event surveillance and drug withdrawals in the United States, 1969–2002: The importance of reporting suspected reactions. Arch. Intern. Med. 165, 1363–1369. https://doi.org/10.1001/archinte.165.12.1363 (2005).
Sgro, C. et al. Incidence of drug-induced hepatic injuries: A French population-based study. Hepatology 36, 451–455. https://doi.org/10.1053/jhep.2002.34857 (2002).
Björnsson, E. S., Bergmann, O. M., Björnsson, H. K., Kvaran, R. B. & Olafsson, S. Incidence, presentation, and outcomes in patients with drug-induced liver injury in the general population of Iceland. Gastroenterology 144, 1419-1425.e1413. https://doi.org/10.1053/j.gastro.2013.02.006 (2013).
Shin, J. et al. Characterizing phenotypes and outcomes of drug-associated liver injury using electronic medical record data. Pharmacoepidemiol. Drug Saf. 22, 190–198. https://doi.org/10.1002/pds.3388 (2013).
Vega, M. et al. The incidence of drug- and herbal and dietary supplement-induced liver injury: Preliminary findings from gastroenterologist-based surveillance in the population of the State of Delaware. Drug Saf. 40, 783–787. https://doi.org/10.1007/s40264-017-0547-9 (2017).
Shen, T. et al. Incidence and etiology of drug-induced liver injury in Mainland China. Gastroenterology 156, 2230-2241.e2211. https://doi.org/10.1053/j.gastro.2019.02.002 (2019).
Schiødt, F. V. et al. Etiology and outcome for 295 patients with acute liver failure in the United States. Liver Transpl. Surg. 5, 29–34. https://doi.org/10.1002/lt.500050102 (1999).
Ostapowicz, G. et al. Results of a prospective study of acute liver failure at 17 tertiary care centers in the United States. Ann. Intern. Med. 137, 947–954. https://doi.org/10.7326/0003-4819-137-12-200212170-00007 (2002).
Russo, M. W., Galanko, J. A., Shrestha, R., Fried, M. W. & Watkins, P. Liver transplantation for acute liver failure from drug induced liver injury in the United States. Liver Transpl. 10, 1018–1023. https://doi.org/10.1002/lt.20204 (2004).
Bernal, W., Auzinger, G., Dhawan, A. & Wendon, J. Acute liver failure. Lancet 376, 190–201. https://doi.org/10.1016/S0140-6736(10)60274-7 (2010).
Chalasani, N. P. et al. ACG Clinical Guideline: The diagnosis and management of idiosyncratic drug-induced liver injury. Am. J. Gastroenterol. 109, 950–966. https://doi.org/10.1038/ajg.2014.131 (2014).
Chalasani, N. & Björnsson, E. Risk factors for idiosyncratic drug-induced liver injury. Gastroenterology 138, 2246–2259. https://doi.org/10.1053/j.gastro.2010.04.001 (2010).
Fontana, R. J. et al. Idiosyncratic drug-induced liver injury is associated with substantial morbidity and mortality within 6 months from onset. Gastroenterology 147, 96-108.e104. https://doi.org/10.1053/j.gastro.2014.03.045 (2014).
Chalasani, N. et al. Features and outcomes of 899 patients with drug-induced liver injury: The DILIN prospective study. Gastroenterology 148, 1340-1352.e1347. https://doi.org/10.1053/j.gastro.2015.03.006 (2015).
Andrade, R. J. et al. Drug-induced liver injury: An analysis of 461 incidences submitted to the spanish registry over a 10-year period. Gastroenterology 129, 512–521. https://doi.org/10.1053/j.gastro.2005.05.006 (2005).
Ball, P., Mandell, L., Niki, Y. & Tillotson, G. Comparative tolerability of the newer fluoroquinolone antibacterials. Drug Saf. 21, 407–421. https://doi.org/10.2165/00002018-199921050-00005 (1999).
Shaw, P. J., Hopfensperger, M. J., Ganey, P. E. & Roth, R. A. Lipopolysaccharide and trovafloxacin coexposure in mice causes idiosyncrasy-like liver injury dependent on tumor necrosis factor-alpha. Toxicol. Sci. 100, 259–266. https://doi.org/10.1093/toxsci/kfm218 (2007).
Sarro, D. A. & Sarro, D. G. Adverse reactions to fluoroquinolones. An overview on mechanistic aspects. Curr. Med. Chem. 8, 371–384. https://doi.org/10.2174/0929867013373435 (2001).
Van Bambeke, F. & Tulkens, P. M. Safety profile of the respiratory fluoroquinolone moxifloxacin. Drug Saf. 32, 359–378. https://doi.org/10.2165/00002018-200932050-00001 (2009).
Olson, H. et al. Concordance of the toxicity of pharmaceuticals in humans and in animals. Regul. Toxicol. Pharmacol. 32, 56–67. https://doi.org/10.1006/rtph.2000.1399 (2000).
Butler, L. D. et al. Current nonclinical testing paradigms in support of safe clinical trials: An IQ Consortium DruSafe perspective. Regul. Toxicol. Pharmacol. 87, S1–S15. https://doi.org/10.1016/j.yrtph.2017.05.009 (2017).
Nguyen, D. G. et al. Bioprinted 3D primary liver tissues allow assessment of organ-level response to clinical drug induced toxicity in vitro. PloS One 11, e0158674. https://doi.org/10.1371/journal.pone.0158674 (2016).
Li, F., Cao, L., Parikh, S. & Zuo, R. Three-dimensional spheroids with primary human liver cells and differential roles of Kupffer cells in drug-induced liver injury. J. Pharm. Sci. 109, 1912–1923. https://doi.org/10.1016/j.xphs.2020.02.021 (2020).
Vernetti, L. A. et al. A human liver microphysiology platform for investigating physiology, drug safety, and disease models. Exp. Biol. Med. (Maywood) 241, 101–114. https://doi.org/10.1177/1535370215592121 (2016).
Ewart, L. et al. Performance assessment and economic analysis of a human liver-chip for predictive toxicology. Commun. Med. 2, 154. https://doi.org/10.1038/s43856-022-00209-1 (2022).
Rennert, K. et al. A microfluidically perfused three dimensional human liver model. Biomaterials 71, 119–131. https://doi.org/10.1016/j.biomaterials.2015.08.043 (2015).
Kaden, T. et al. Generation & characterization of expandable human liver sinusoidal endothelial cells and their application to assess hepatotoxicity in an advanced in vitro liver model. Toxicology 483, 153374. https://doi.org/10.1016/j.tox.2022.153374 (2023).
Spiess, M. The asialoglycoprotein receptor: A model for endocytic transport receptors. Biochemistry 29, 10009–10018. https://doi.org/10.1021/bi00495a001 (1990).
Shi, B., Abrams, M. & Sepp-Lorenzino, L. Expression of asialoglycoprotein receptor 1 in human hepatocellular carcinoma. J. Histochem. Cytochem. 61, 901–909. https://doi.org/10.1369/0022155413503662 (2013).
Cerec, V. et al. Transdifferentiation of hepatocyte-like cells from the human hepatoma HepaRG cell line through bipotent progenitor. Hepatology 45, 957–967. https://doi.org/10.1002/hep.21536 (2007).
Hayes, J. D., Flanagan, J. U. & Jowsey, I. R. Glutathione transferases. Annu. Rev. Pharmacol. Toxicol. 45, 51–88. https://doi.org/10.1146/annurev.pharmtox.45.120403.095857 (2004).
Smedsrød, B. Clearance function of scavenger endothelial cells. Comp. Hepatol. 3(Suppl 1), S22–S22. https://doi.org/10.1186/1476-5926-2-S1-S22 (2004).
Taylor, P. R. et al. Macrophage receptors and immune recognition. Annu. Rev. Immunol. 23, 901–944. https://doi.org/10.1146/annurev.immunol.23.021704.115816 (2004).
Shaw, P. J., Ganey, P. E. & Roth, R. A. Idiosyncratic drug-induced liver injury and the role of inflammatory stress with an emphasis on an animal model of trovafloxacin hepatotoxicity. Toxicol. Sci. 118, 7–18. https://doi.org/10.1093/toxsci/kfq168 (2010).
Hsiao, C.-J.J., Younis, H. & Boelsterli, U. A. Trovafloxacin, a fluoroquinolone antibiotic with hepatotoxic potential, causes mitochondrial peroxynitrite stress in a mouse model of underlying mitochondrial dysfunction. Chem. Biol. Interact. 188, 204–213. https://doi.org/10.1016/j.cbi.2010.07.017 (2010).
Ito, Y., Bethea, N. W., Abril, E. R. & McCuskey, R. S. Early hepatic microvascular injury in response to acetaminophen toxicity. Microcirculation 10, 391–400. https://doi.org/10.1038/sj.mn.7800204 (2003).
Kostadinova, R. et al. A long-term three dimensional liver co-culture system for improved prediction of clinically relevant drug-induced hepatotoxicity. Toxicol. Appl. Pharmacol. 268, 1–16. https://doi.org/10.1016/j.taap.2013.01.012 (2013).
Waring, J. F. et al. Microarray analysis of lipopolysaccharide potentiation of trovafloxacin-induced liver injury in rats suggests a role for proinflammatory chemokines and neutrophils. J. Pharmacol. Exp. Ther. 316, 1080. https://doi.org/10.1124/jpet.105.096347 (2006).
Shaw, P. J. et al. Coexposure of mice to trovafloxacin and lipopolysaccharide, a model of idiosyncratic hepatotoxicity, results in a unique gene expression profile and interferon gamma-dependent liver injury. Toxicol. Sci. 107, 270–280. https://doi.org/10.1093/toxsci/kfn205 (2009).
Shaw, P. J. et al. Trovafloxacin enhances TNF-induced inflammatory stress and cell death signaling and reduces TNF clearance in a murine model of idiosyncratic hepatotoxicity. Toxicol. Sci. 111, 288–301. https://doi.org/10.1093/toxsci/kfp163 (2009).
Shaw, P. J., Ganey, P. E. & Roth, R. A. Tumor necrosis factor alpha is a proximal mediator of synergistic hepatotoxicity from trovafloxacin/lipopolysaccharide coexposure. J. Pharmacol. Exp. Ther. 328, 62–68. https://doi.org/10.1124/jpet.108.143792 (2009).
Giustarini, G. et al. Tissue influx of neutrophils and monocytes is delayed during development of trovafloxacin-induced tumor necrosis factor-dependent liver injury in mice. J. Appl. Toxicol. 38, 753–765. https://doi.org/10.1002/jat.3585 (2018).
Giustarini, G. et al. Trovafloxacin-induced liver injury: Lack in regulation of inflammation by inhibition of nucleotide release and neutrophil movement. Toxicol Sci 167, 385–396. https://doi.org/10.1093/toxsci/kfy244 (2019).
Poon, I. K. H. et al. Unexpected link between an antibiotic, pannexin channels and apoptosis. Nature 507, 329–334. https://doi.org/10.1038/nature13147 (2014).
Giustarini, G. et al. The hepatotoxic fluoroquinolone trovafloxacin disturbs TNF- and LPS-induced p65 nuclear translocation in vivo and in vitro. Toxicol. Appl. Pharmacol. 391, 114915. https://doi.org/10.1016/j.taap.2020.114915 (2020).
Bradham, C. A., Plümpe, J., Manns, M. P., Brenner, D. A. & Trautwein, C. I. TNF-induced liver injury. Am. J. Physiol. Gastrointest. Liver Physiol. 275, 387–392. https://doi.org/10.1152/ajpgi.1998.275.3.G387 (1998).
Woolbright, B. L. & Jaeschke, H. Mechanisms of inflammatory liver injury and drug-induced hepatotoxicity. Curr. Pharmacol. Rep. 4, 346–357. https://doi.org/10.1007/s40495-018-0147-0 (2018).
Bonzo, J. A. et al. Differential effects of trovafloxacin on TNF-α and IL-6 profiles in a rat hepatocyte-Kupffer cell coculture system. Appl. Vitro Toxicol. 1, 45–54. https://doi.org/10.1089/aivt.2014.0004 (2015).
Schmidt-Arras, D. & Rose-John, S. IL-6 pathway in the liver: From physiopathology to therapy. J. Hepatol. 64, 1403–1415. https://doi.org/10.1016/j.jhep.2016.02.004 (2016).
Masubuchi, Y. et al. Role of interleukin-6 in hepatic heat shock protein expression and protection against acetaminophen-induced liver disease. Biochem. Biophys. Res. Commun. 304, 207–212. https://doi.org/10.1016/S0006-291X(03)00572-2 (2003).
Kovalovich, K. et al. Increased toxin-induced liver injury and fibrosis in interleukin-6–deficient mice. Hepatology 31, 149–159. https://doi.org/10.1002/hep.510310123 (2000).
Feder, L. S., Todaro, J. A. & Laskin, D. L. Characterization of interleukin-1 and interleukin-6 production by hepatic endothelial cells and macrophages. J. Leukoc. Biol. 53, 126–132. https://doi.org/10.1002/jlb.53.2.126 (1993).
Vidal-Vanaclocha, F. et al. IL-18 regulates IL-1β-dependent hepatic melanoma metastasis via vascular cell adhesion molecule-1. Proc. Natl. Acad. Sci. 97, 734–739. https://doi.org/10.1073/pnas.97.2.734 (2000).
Wu, J. et al. Toll-like receptor-induced innate immune responses in non-parenchymal liver cells are cell type-specific. Immunology 129, 363–374. https://doi.org/10.1111/j.1365-2567.2009.03179.x (2010).
Wang, Y., Zhang, Y., Liu, Y., Xu, J. & Liu, Y. Gut-liver axis: Liver sinusoidal endothelial cells function as the hepatic barrier in colitis-induced liver injury. Front. Cell Dev. Biol. 9, 702890. https://doi.org/10.3389/fcell.2021.702890 (2021).
Dong, W. et al. Toxic metals stimulate inflammatory cytokines in hepatocytes through oxidative stress mechanisms. Toxicol. Appl. Pharmacol. 151, 359–366. https://doi.org/10.1006/taap.1998.8481 (1998).
Thornton, A. J., Strieter, R. M., Lindley, I., Baggiolini, M. & Kunkel, S. L. Cytokine-induced gene expression of a neutrophil chemotactic factor/IL-8 in human hepatocytes. J. Immunol. 144, 2609 (1990).
González-Amaro, R. et al. Induction of tumor necrosis factor alpha production by human hepatocytes in chronic viral hepatitis. J. Exp. Med. 179, 841–848. https://doi.org/10.1084/jem.179.3.841 (1994).
Saad, B., Frei, K., Scholl, F. A., Fontana, A. & Maier, P. Hepatocyte-derived Interleukin-6 and tumor-necrosis factor α mediate the lipopolysaccharide-induced acute-phase response and nitric oxide release by cultured rat hepatocytes. Eur. J. Biochem. 229, 349–355. https://doi.org/10.1111/j.1432-1033.1995.0349k.x (1995).
Tanaka, Y., Yamato, M., Okano, T., Kitamori, T. & Sato, K. Evaluation of effects of shear stress on hepatocytes by a microchip-based system. Meas. Sci. Technol. 17, 3167. https://doi.org/10.1088/0957-0233/17/12/S08 (2006).
DeLeve, L. D., Wang, X., Hu, L., McCuskey, M. K. & McCuskey, R. S. Rat liver sinusoidal endothelial cell phenotype is maintained by paracrine and autocrine regulation. Am. J. Physiol. Gastrointest. Liver Physiol. 287, G757–G763. https://doi.org/10.1152/ajpgi.00017.2004 (2004).
Liguori, M. J. et al. Microarray analysis in human hepatocytes suggests a mechanism for hepatotoxicity induced by trovafloxacin. Hepatology 41, 177–186. https://doi.org/10.1002/hep.20514 (2005).
Guillouzo, A. et al. The human hepatoma HepaRG cells: A highly differentiated model for studies of liver metabolism and toxicity of xenobiotics. Chem. Biol. Interact. 168, 66–73. https://doi.org/10.1016/j.cbi.2006.12.003 (2007).
Kanebratt, K. P. & Andersson, T. B. Evaluation of HepaRG cells as an in vitro model for human drug metabolism studies. Drug Metab. Dispos. 36, 1444. https://doi.org/10.1124/dmd.107.020016 (2008).
Lübberstedt, M. et al. HepaRG human hepatic cell line utility as a surrogate for primary human hepatocytes in drug metabolism assessment in vitro. J. Pharmacol. Toxicol. Methods 63, 59–68. https://doi.org/10.1016/j.vascn.2010.04.013 (2011).
Nelson, L. J. et al. Human hepatic HepaRG cells maintain an organotypic phenotype with high intrinsic CYP450 activity/metabolism and significantly outperform standard HepG2/C3A cells for pharmaceutical and therapeutic applications. Basic Clin. Pharmacol. Toxicol. 120, 30–37. https://doi.org/10.1111/bcpt.12631 (2017).
Fraczek, J., Bolleyn, J., Vanhaecke, T., Rogiers, V. & Vinken, M. Primary hepatocyte cultures for pharmaco-toxicological studies: At the busy crossroad of various anti-dedifferentiation strategies. Arch. Toxicol. 87, 577–610. https://doi.org/10.1007/s00204-012-0983-3 (2013).
Elvevold, K., Smedsrød, B. & Martinez, I. The liver sinusoidal endothelial cell: A cell type of controversial and confusing identity. Am. J. Physiol. Gastrointest. Liver Physiol. 294, G391–G400. https://doi.org/10.1152/ajpgi.00167.2007 (2008).
Heuff, G., Van Loosdrecht, A. A. D., Betjes, M. G. H., Beelen, R. H. J. & Meijer, S. Isolation and purification of large quantities of fresh human Kupffer cells, which are cytotoxic against colon carcinoma. Hepatology 21, 740–745. https://doi.org/10.1002/hep.1840210322 (1995).
Raasch, M., Fritsche, E., Kurtz, A., Bauer, M. & Mosig, A. S. Microphysiological systems meet hiPSC technology—New tools for disease modeling of liver infections in basic research and drug development. Adv. Drug Deliv. Rev. 140, 51–67. https://doi.org/10.1016/j.addr.2018.06.008 (2019).
Gripon, P. et al. Infection of a human hepatoma cell line by hepatitis B virus. Proc. Natl. Acad. Sci. 99, 15655–15660. https://doi.org/10.1073/pnas.232137699 (2002).
Kmiec, Z. Cooperation of liver cells in health and disease. Adv. Anat. Embryol. Cell Boil. 161, III–XIII, 1, Doi: https://doi.org/10.1007/978-3-642-56553-3_7 (2001).
Lalor, P. F. & Adams, D. H. Adhesion of lymphocytes to hepatic endothelium. Mol. Pathol. 52, 214–219. https://doi.org/10.1136/mp.52.4.214 (1999).
Rashidi, H., Alhaque, S., Szkolnicka, D., Flint, O. & Hay, D. C. Fluid shear stress modulation of hepatocyte-like cell function. Arch. Toxicol. 90, 1757–1761. https://doi.org/10.1007/s00204-016-1689-8 (2016).
Xu, J. J. et al. Cellular imaging predictions of clinical drug-induced liver injury. Toxicol. Sci. 105, 97–105. https://doi.org/10.1093/toxsci/kfn109 (2008).
Fish, D. N. & Chow, A. T. The clinical pharmacokinetics of levofloxacin. Clin. Pharmacokinet. 32, 101–119. https://doi.org/10.2165/00003088-199732020-00002 (1997).
Vincent, J., Teng, R., Dalvie, D. K. & Friedman, H. L. Pharmacokinetics and metabolism of single oral doses of trovafloxacin. Am. J. Surg. 176, 8S-13S. https://doi.org/10.1016/S0002-9610(98)00213-X (1998).
Teng, R. et al. Pharmacokinetics and safety of trovafloxacin (CP-99, 219), a new quinolone antibiotic, following adminstration of single oral doses to healthy male volunteers. J. Antimicrob. Chemother. 36, 385–394. https://doi.org/10.1093/jac/36.2.385 (1995).
Lubasch, A., Keller, I., Borner, K., Koeppe, P. & Lode, H. Comparative pharmacokinetics of ciprofloxacin, gatifloxacin, grepafloxacin, levofloxacin, trovafloxacin, and moxifloxacin after single oral administration in healthy volunteers. Antimicrob. Agents Chemother. 44, 2600–2603. https://doi.org/10.1128/AAC.44.10.2600-2603.2000 (2000).
- SEO Powered Content & PR Distribution. Get Amplified Today.
- PlatoData.Network Vertical Generative Ai. Empower Yourself. Access Here.
- PlatoAiStream. Web3 Intelligence. Knowledge Amplified. Access Here.
- PlatoESG. Automotive / EVs, Carbon, CleanTech, Energy, Environment, Solar, Waste Management. Access Here.
- PlatoHealth. Biotech and Clinical Trials Intelligence. Access Here.
- ChartPrime. Elevate your Trading Game with ChartPrime. Access Here.
- BlockOffsets. Modernizing Environmental Offset Ownership. Access Here.
- Source: https://www.nature.com/articles/s41598-023-40004-z