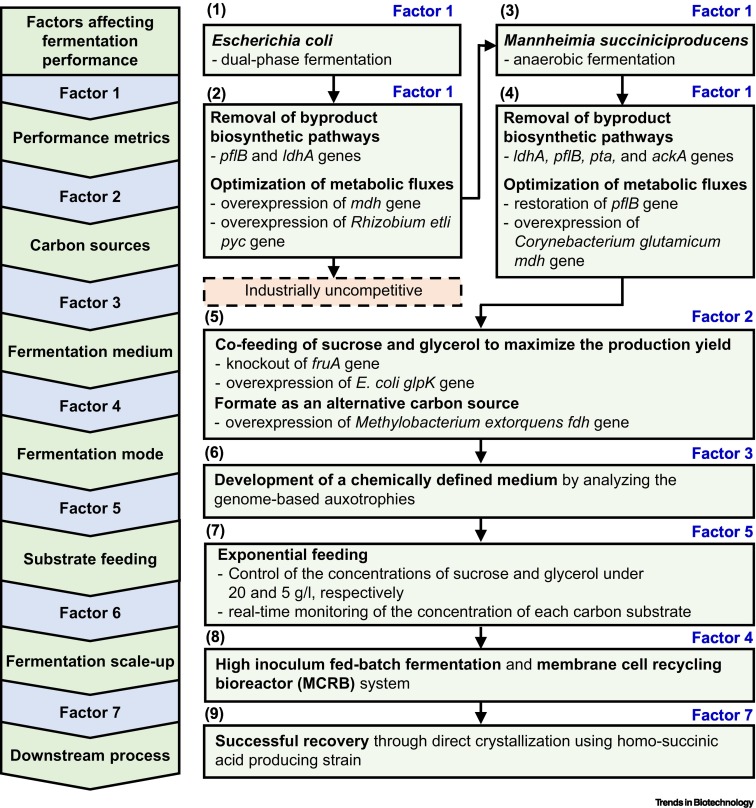
Tools and strategies of systems metabolic engineering for the development of microbial cell factories for chemical production.
Chem. Soc. Rev. 2020; 49: 4615-4636
Is net zero carbon 2050 possible?.
Joule. 2020; 4: 2237-2240
Insights into the European Market of Bio-based Chemicals.
Publications Office of the European Union, 2019
US Biobased Products Market Potential and Projections through 2025.
Nova Science Publishers, 2010
Metabolic engineering of Escherichia coli for natural product biosynthesis.
Trends Biotechnol. 2020; 38: 745-765
Metabolic engineering strategies toward production of biofuels.
Curr. Opin. Chem. Biol. 2020; 59: 1-14
Systems metabolic engineering strategies: integrating systems and synthetic biology with metabolic engineering.
Trends Biotechnol. 2019; 37: 817-837
On the future fermentation.
Microb. Biotechnol. 2021; 14: 18-21
High cell-density culture of Escherichia coli.
Trends Biotechnol. 1996; 14: 98-105
Enhanced succinic acid production by Mannheimia employing optimal malate dehydrogenase.
Nat. Commun. 2020; 11: 1970
Glutaric acid production by systems metabolic engineering of an l-lysine–overproducing Corynebacterium glutamicum.
Proc. Natl. Acad. Sci. U. S. A. 2020; 117: 30328-30334
Advances in bioconversion of glycerol to 1, 3-propanediol: prospects and challenges.
Process Biochem. 2018; 71: 134-146
Development of a commercial scale process for production of 1, 4-butanediol from sugar.
Curr. Opin. Biotechnol. 2016; 42: 118-125
Impact of synthetic biology and metabolic engineering on industrial production of fine chemicals.
Biotechnol. Adv. 2015; 33: 1395-1402
Synthetic biology’s first malaria drug meets market resistance.
Nature. 2016; 530: 389
Bio-vanillin: towards a sustainable industrial production.
Trends Food Sci. Technol. 2021; 109: 579-592
Microbial polyhydroxyalkanoates and nonnatural polyesters.
Adv. Mater. 2020; 32: 1907138
Systems metabolic engineering strategies for non-natural microbial polyester production.
Biotechnol. J. 2019; 14: 1800426
One-step fermentative production of poly (lactate-co-glycolate) from carbohydrates in Escherichia coli.
Nat. Biotechnol. 2016; 34: 435-440
Comparative efficiency and driving range of light-and heavy-duty vehicles powered with biomass energy stored in liquid fuels or batteries.
Proc. Natl. Acad. Sci. U. S. A. 2014; 111: 3360-3364
From the first drop to the first truckload: commercialization of microbial processes for renewable chemicals.
Curr. Opin. Biotechnol. 2013; 24: 1061-1068
Model-driven evaluation of the production potential for growth-coupled products of Escherichia coli.
Metab. Eng. 2010; 12: 173-186
A molecular switch that enhances productivity of bioprocesses for heterologous metabolite production.
Mol. Syst. Des. Eng. 2018; 3: 550-559
Dynamic strain scanning optimization: an efficient strain design strategy for balanced yield, titer, and productivity. DySScO strategy for strain design.
BMC Biotechnol. 2013; 13: 8
Novel two-stage processes for optimal chemical production in microbes.
Metab. Eng. 2020; 62: 186-197
The bioprocess TEA calculator: an online technoeconomic analysis tool to evaluate the commercial competitiveness of potential bioprocesses.
Metab. Eng. 2021; 65: 42-51
The emergence of adaptive laboratory evolution as an efficient tool for biological discovery and industrial biotechnology.
Metab. Eng. 2019; 56: 1-16
In situ recovery of fermentation products.
Trends Biotechnol. 1984; 2: 129-136
In situ recovery of bio-based carboxylic acids.
Green Chem. 2018; 20: 1791-1804
Systems strategies for developing industrial microbial strains.
Nat. Biotechnol. 2015; 33: 1061-1072
Evaluation of different biomass materials as feedstock for fermentable sugar production.
Appl. Biochem. Biotechnol. 2007; 137: 423-435
From lignin to nylon: cascaded chemical and biochemical conversion using metabolically engineered Pseudomonas putida.
Metab. Eng. 2018; 47: 279-293
Consolidated bioprocessing for bioethanol production by metabolically engineered Bacillus subtilis strains.
Sci. Rep. 2021; 11: 13731
Recent insights into consolidated bioprocessing for lignocellulosic biohydrogen production.
Int. J. Hydrog. Energy. 2019; 44: 14362-14379
Consolidated bioprocessing performance of a two-species microbial consortium for butanol production from lignocellulosic biomass.
Biotechnol. Bioeng. 2020; 117: 2985-2995
Consolidated bioprocessing performance of bacterial consortium EMSD5 on hemicellulose for isopropanol production.
Bioresour. Technol. 2019; 292121965
Consolidated bioprocessing of lignocellulose for production of glucaric acid by an artificial microbial consortium.
Biotechnol. Biofuels. 2021; 14: 110
Fermentation of glycerol and production of valuable chemical and biofuel molecules.
Biotechnol. Lett. 2013; 35: 831-842
Bioconversion technologies of crude glycerol to value added industrial products.
Biotechnol. Rep. 2016; 9: 9-14
Resource recovery and circular economy from organic solid waste using aerobic and anaerobic digestion technologies.
Bioresour. Technol. 2020; 301122778
Designing and engineering Methylorubrum extorquens AM1 for itaconic acid production.
Front. Microbiol. 2019; 10: 1027
Metabolic engineering of Methylobacterium extorquens AM1 for the production of butadiene precursor.
Microb. Cell Factories. 2018; 17: 194
Engineering Methylobacterium extorquens for de novo synthesis of the sesquiterpenoid α-humulene from methanol.
Metab. Eng. 2015; 32: 82-94
Microbial production of poly (hydroxybutyrate) from C 1 carbon sources.
Appl. Microbiol. Biotechnol. 2013; 97: 1407-1424
Synthetic formatotrophs for one-carbon biorefinery.
Adv. Sci. 2021; 8: 2100199
Escherichia coli is engineered to grow on CO2 and formic acid.
Nat. Microbiol. 2020; 5: 1459-1463
Renewable methanol and formate as microbial feedstocks.
Curr. Opin. Biotechnol. 2020; 62: 168-180
Metabolic engineering of Corynebacterium glutamicum for L-arginine production.
Nat. Commun. 2014; 5: 4618
Formic acid as a secondary substrate for succinic acid production by metabolically engineered Mannheimia succiniciproducens.
Biotechnol. Bioeng. 2017; 114: 2837-2847
Escherichia coli W as a new platform strain for the enhanced production of L-valine by systems metabolic engineering.
Biotechnol. Bioeng. 2011; 108: 1140-1147
High-yield production of L-valine in engineered Escherichia coli by a novel two-stage fermentation.
Metab. Eng. 2020; 62: 198-206
Increase of lycopene production by supplementing auxiliary carbon sources in metabolically engineered Escherichia coli.
Appl. Microbiol. Biotechnol. 2011; 90: 489-497
Enhanced 1, 3-propanediol production by supply of organic acids and repeated fed-batch culture.
J. Ind. Microbiol. Biotechnol. 2010; 37: 681-687
Efficient production of 1, 3-propanediol from crude glycerol by repeated fed-batch fermentation strategy of a lactate and 2, 3-butanediol deficient mutant of Klebsiella pneumoniae.
Microb. Cell Factories. 2018; 17: 92
Strategies for fermentation medium optimization: an in-depth review.
Front. Microbiol. 2017; 7: 2087
Large-scale production of enzymes for biotechnology uses.
Curr. Opin. Biotechnol. 2021; 69: 68-76
Plasmid addiction systems: perspectives and applications in biotechnology.
Microb. Biotechnol. 2010; 3: 634-657
Techniques for chromosomal integration and expression optimization in Escherichia coli.
Biotechnol. Bioeng. 2018; 115: 2467-2478
Effect of genomic integration location on heterologous protein expression and metabolic engineering in E. coli.
ACS Synth. Biol. 2017; 6: 710-720
Metabolic engineering of Escherichia coli for high-level astaxanthin production with high productivity.
Metab. Eng. 2018; 49: 105-115
Current status and applications of adaptive laboratory evolution in industrial microorganisms.
J. Microbiol. Biotechnol. 2020; 30: 793-803
Tolerance improvement of Corynebacterium glutamicum on lignocellulose derived inhibitors by adaptive evolution.
Appl. Microbiol. Biotechnol. 2018; 102: 377-388
Stress-tolerant non-conventional microbes enable next-generation chemical biosynthesis.
Nat. Chem. Biol. 2020; 16: 113-121
Muconic acid production from glucose and xylose in Pseudomonas putida via evolution and metabolic engineering.
Nat. Commun. 2022; 13: 4925
Sea water as a reaction medium for bioethanol production.
in: Patra J. Microbial Biotechnology. Springer, 2018: 171-192
Halophiles and their vast potential in biofuel production.
Front. Microbiol. 2019; 10: 1895
Halophiles, coming stars for industrial biotechnology.
Biotechnol. Adv. 2015; 33: 1433-1442
The establishment of a marine focused biorefinery for bioethanol production using seawater and a novel marine yeast strain.
Sci. Rep. 2018; 8: 12127
Non-sterilized fermentation of 2,3-butanediol with seawater by metabolic engineered fast-growing Vibrio natriegens.
Front. Bioeng. Biotechnol. 2022; 10955097
Rational flux-tuning of Halomonas bluephagenesis for co-production of bioplastic PHB and ectoine.
Nat. Commun. 2020; 11: 3313
Stoichiometric and kinetic analysis of extreme halophilic Archaea on various substrates in a corrosion resistant bioreactor.
New Biotechnol. 2014; 31: 80-89
A beginner’s guide to bioprocess modes-batch, fed-batch, and continuous fermentation.
Application Notes-Eppendorf. 2019; 408: 1-16
A review of control strategies for manipulating the feed rate in fed-batch fermentation processes.
J. Biotechnol. 2017; 245: 34-46
Open and continuous fermentation: products, conditions and bioprocess economy.
Biotechnol. J. 2014; 9: 1503-1511
Development of a cell-recycled continuous fermentation process for enhanced production of succinic acid by high-yielding mutants of Actinobacillus succinogenes.
Biotechnol. Bioprocess Eng. 2021; 26: 125-136
Homo-succinic acid production by metabolically engineered Mannheimia succiniciproducens.
Metab. Eng. 2016; 38: 409-417
Improve gamma-aminobutyric acid production in Corynebacterium glutamicum by optimizing the metabolic flux.
Syst. Microbiol. Biomanuf. 2022; 2: 305-316
Designing an irreversible metabolic switch for scalable induction of microbial chemical production.
Nat. Commun. 2021; 12: 3419
Systematic engineering of TCA cycle for optimal production of a four-carbon platform chemical 4-hydroxybutyric acid in Escherichia coli.
Metab. Eng. 2016; 38: 264-273
Production of succinic acid by metabolically engineered microorganisms.
Curr. Opin. Biotechnol. 2016; 42: 54-66
Production of succinic acid by engineered E. coli strains using soybean carbohydrates as feedstock under aerobic fermentation conditions.
Bioresour. Technol. 2013; 130: 398-405
Metabolic engineering of Escherichia coli for the production of succinate from glycerol.
Metab. Eng. 2010; 12: 409-419
Metabolic impact of the level of aeration during cell growth on anaerobic succinate production by an engineered Escherichia coli strain.
Metab. Eng. 2010; 12: 499-509
Current advance in biological production of malic acid using wild type and metabolic engineered strains.
Bioresour. Technol. 2018; 258: 345-353
Combining rational metabolic engineering and flux optimization strategies for efficient production of fumaric acid.
Appl. Microbiol. Biotechnol. 2015; 99: 8455-8464
Oxidative stress-related responses of Bifidobacterium longum subsp. longum BBMN68 at the proteomic level after exposure to oxygen.
Microbiology. 2011; 157: 1573-1588
Microaerobic fermentation of Lactobacillus acidophilus within gut microbiome physiological conditions by BioFlo® bioprocess control stations.
Application Notes-Eppendorf. 2019; 412: 1-8
Automated measurement and monitoring of bioprocesses: key elements of the M3C strategy.
in: Mandenius C.F. Titchener-Hooker N. Measurement, Monitoring, Modelling and Control of Bioprocesses. Springer, 2012: 1-33
Succinic acid production from glycerol by Actinobacillus succinogenes using dimethylsulfoxide as electron acceptor.
New Biotechnol. 2014; 31: 133-139
Metabolic engineering of Escherichia coli for the enhanced production of l-tyrosine.
Biotechnol. Bioeng. 2018; 115: 2554-2564
Bioprocessing in the digital age: the role of process models.
Biotechnol. J. 2020; 15: 1900172
Nonlinear model predictive control of fed-batch fermentations using dynamic flux balance models.
J. Process Control. 2016; 42: 137-149
Real-time monitoring of bacterial growth kinetics in suspensions using laser speckle imaging.
Sci. Rep. 2020; 10: 408
On-line analysis and in situ pH monitoring of mixed acid fermentation by Escherichia coli using combined FTIR and Raman techniques.
Anal. Bioanal. Chem. 2020; 412: 7307-7319
Scale-up of industrial microbial processes.
FEMS Microbiol. Lett. 2018; 365: fny138
A model-based framework for parallel scale-down fed-batch cultivations in mini-bioreactors for accelerated phenotyping.
Biotechnol. Bioeng. 2019; 116: 2906-2918
Developing a computational framework to advance bioprocess scale-up.
Trends Biotechnol. 2020; 38: 846-856
Reproduction of large-scale bioreactor conditions on microfluidic chips.
Microorganisms. 2019; 7: 105
Development of dynamic compartment models for industrial aerobic fed-batch fermentation processes.
Chem. Eng. J. 2021; 420130402
Grand research challenges for sustainable industrial biotechnology.
Trends Biotechnol. 2019; 37: 1042-1050
Engineering thermophilic Geobacillus thermoglucosidasius for riboflavin production.
Microb. Biotechnol. 2021; 14: 363-373
Metabolic engineering of microbial competitive advantage for industrial fermentation processes.
Science. 2016; 353: 583-586
Synthesis and analysis of separation processes for extracellular chemicals generated from microbial conversions.
BMC Chem. Eng. 2019; 1: 21
Synthesis and analysis of separation networks for the recovery of intracellular chemicals generated from microbial-based conversions.
Biotechnol. Biofuels. 2017; 10: 119
Metabolic engineering of Escherichia coli for secretory production of free haem.
Nat. Catal. 2018; 1: 720-728
Microbial production of methyl anthranilate, a grape flavor compound.
Proc. Natl. Acad. Sci. U. S. A. 2019; 116: 10749-10756
Engineering of unconventional yeast Yarrowia lipolytica for efficient succinic acid production from glycerol at low pH.
Metab. Eng. 2017; 42: 126-133
Monascus ruber as cell factory for lactic acid production at low pH.
Metab. Eng. 2017; 42: 66-73
Machine learning applications in systems metabolic engineering.
Curr. Opin. Biotechnol. 2020; 64: 1-9
Optimizing chemical reactions with deep reinforcement learning.
ACS Cent. Sci. 2017; 3: 1337-1344
Systematic strategies for developing phage resistant Escherichia coli strains.
Nat. Commun. 2022; 13: 4491
Succinate production in dual-phase Escherichia coli fermentations depends on the time of transition from aerobic to anaerobic conditions.
J. Ind. Microbiol. Biotechnol. 2002; 28: 325-332
Genome-based metabolic engineering of Mannheimia succiniciproducens for succinic acid production.
Appl. Environ. Microbiol. 2006; 72: 1939-1948
Development of chemically defined medium for Mannheimia succiniciproducens based on its genome sequence.
Appl. Microbiol. Biotechnol. 2008; 79: 263-272
Highly selective production of succinic acid by metabolically engineered Mannheimia succiniciproducens and its efficient purification.
Biotechnol. Bioeng. 2016; 113: 2168-2177
Synthesis, characterization, and application of fully biobased and biodegradable nylon-4, 4 and-5, 4.
ACS Sustain. Chem. Eng. 2020; 8: 5604-5614
Challenges and opportunities in bringing nonbiological atoms to life with synthetic metabolism.
Trends Biotechnol. 2022; ()
Recombinant Escherichia coli as a biofactory for various single-and multi-element nanomaterials.
Proc. Natl. Acad. Sci. U. S. A. 2018; 115: 5944-5949
Biosynthesis of inorganic nanomaterials using microbial cells and bacteriophages.
Nat. Rev. Chem. 2020; 4: 638-656
- Coinsmart. Europe’s Best Bitcoin and Crypto Exchange.Click Here
- Platoblockchain. Web3 Metaverse Intelligence. Knowledge Amplified. Access Here.
- Source: https://www.cell.com/trends/biotechnology/fulltext/S0167-7799(22)00276-1?rss=yes